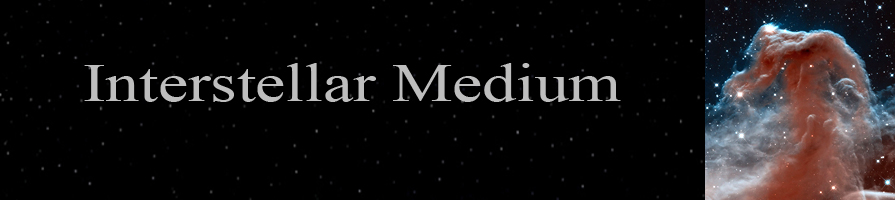
Interstellar Medium (ISM) Overview
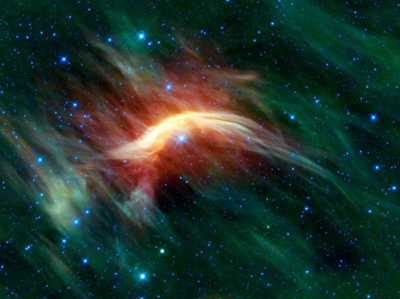
The Interstellar Medium. One tends to think of outer space as consisting of stars separated by great distances with a vacuum between them. While it is true that space is mostly empty, there are regions that contain considerable amounts of material. This is relative of course. By earthly standards such regions would still constitute a pretty good vacuum. The Interstellar Medium consists of all the materials that fill the space between the stars, which is mainly gas (99%) and a small amount of dust (1%). In total, about 15% of the visible matter in the Milky Way is composed of interstellar gas and dust.
Observe the Bow Shock Nebula to the left. Nebula is a Latin word for cloud and "nebula" is used as a generic term for regions of intense interstellar gas and/or dust clouds.
The typical gas density in space is one atom per cubic centimeter (cc). The best man-made vacuum is about a 10^12 atoms per cubic centimeter. A cubic centimeter of air in a normal room has about 10^19 atoms in it. The interstellar medium is not uniform in density. Although on average its density is one atom per cc, a nebula can have densities up to a million atoms per cc.
Interstellar gas is composed of either molecules or atoms of gasses with hydrogen and helium being the most abundant. Carbon monoxide, oxygen and nitrogen are other forms of interstellar gas, but in much, much smaller quantities. Dust is composed of little bits of solid matter. Dust is very small, on the order of microns (10^-6 meters). Dust is mainly made of compounds of carbon and silicon in various forms and out of various forms of ice such as water, carbon dioxide, and ammonia (graphite grains, silicate grains, etc.). Temperatures have to be pretty low to have ice particles - 100 K and below. Other kinds of dust can exist at higher temperatures, but if temperatures gets too high, all the different kinds of dust are decimated. The interstellar composition, structure, etc. of dust particles remains an area of some uncertainty in astro-physics.
The interstellar medium can be divided into three different categories dependent on their individual temperatures: cold (10 to 99 Kelvin), warm (100 to 1,000's K) and hot (millions K). The colder a cloud of gas, the more of its output emission is in the long wavelengths; radio and microwave. Hot regions of interstellar gas are bright in their own material optical emissions. Note also that the various temperatures determine the type of matter that will exist. Cold temperatures are suitable to the formation of molecules. Warmer temperatures will find only atoms, such as neutral hydrogen. Under higher temperatures, atoms become ionized. The coldest regions of space are the dark nebula. Top
Interstellar Gas
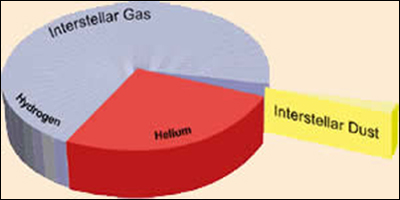
About 75% of the Interstellar Medium is in the form of hydrogen with about 24% helium. The interstellar gas consists partly of neutral atoms and molecules, as well as charged particles such as ions and electrons. The gas is extremely dilute with an average density of 1 atom per cubic centimeter (cc). (For comparison, the air we breathe has a density of approximately 3 x 10^19 molecules per cc.) Even though the interstellar gas is very dilute, the total amount of matter adds up because of the vast distances between the stars.
Interstellar gas is typically found in one of two different formulations:
- Cold clouds of neutral atomic or molecular hydrogen.
- Hot ionized hydrogen near hot young stars.
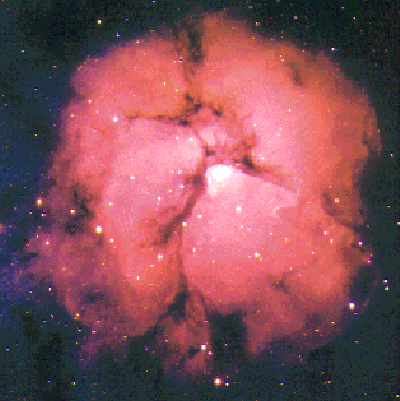
The power to keep an emission nebula glowing is provided by hot, high energy stars in the interior of the nebula, specifically class O (letter O) and class B1 stars, the hottest star categories. The ionization of hydrogen and helium requires high energy ultraviolet photons such as those that are emitted from these types of stars.
The cold clouds of neutral or molecular hydrogen are the birthplace of new stars as they become gravitationally unstable and collapse. The neutral and molecular forms emit radiation in the radio band of the electro-magnetic spectrum.
Ionized hydrogen is produced when large amounts of ultraviolet radiation are released by hot newly-formed stars. This radiation ionizes the surrounding clouds of gas. Visible light is emitted when electrons recombine with the ionized hydrogen, which is seen as beautiful red colors of "emission nebula".
See the Trifid Nebula (M20) image to the left. The red light comes from hydrogen emission, excited by the ultraviolet radiation from hot stars embedded in the nebula. The dark regions criss-crossing the nebula are obscuring dust.
Emission nebula are also known as H II (H-two) regions. The explanation of this nomenclature is: The H means Hydrogen and the Roman numeral II means that the hydrogen is ionized (its electron has been stripped off by high energy photons). H I (H-one) is neutral hydrogen meaning that it still has its electron. He II would be singly ionized helium, and He III would be doubly (fully) ionized helium.
Astronomers had predicted that molecular oxygen would be the third most common interstellar molecule, after molecular hydrogen (H II) and carbon monoxide (CO). But the experimental evidence found that the oxygen molecule is much rarer than theory predicts. For example, hydrogen molecules in the Orion Nebula outnumber oxygen molecules a million to one. To explain this scarcity, astronomers recently proposed that oxygen atoms might bind tightly to dust particles that are found in most outer space clouds.
To see how readily oxygen atoms can escape, scientists heated two types of solids that make up interstellar dust grains - water ice and silicate. They found that the binding energy of oxygen is more than twice what had been previously estimated - 0.14 electron volts for water ice and 0.16 electron volts for silicate. These binding energies are high enough to keep oxygen atoms stuck to stardust. But when the oxygen atoms stay stuck to the dust grains, hydrogen atoms combine with the oxygen to create water ice (H2O) instead. The water can then become part of asteroids, comets, and planets, setting the stage for the creation of life. Top
Neutral Hydrogen Emits Some Radiation!
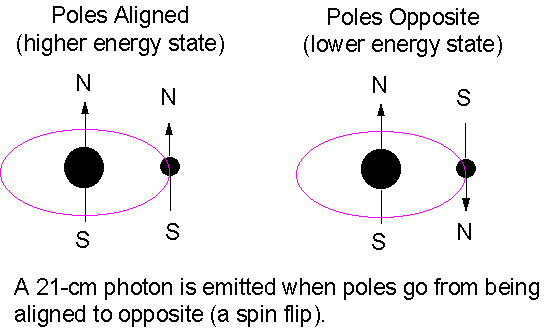
The Milky Way Galaxy is filled with a very diffuse distribution of neutral hydrogen gas. The interstellar medium is far too cool to excite the higher energy states of hydrogen, but there is a feature at the 21 centimeter (cm) wavelength in the radio frequency. 21-cm photons are produced by the spinning magnetic fields of the hydrogen atom's nuclear proton and orbiting electron.
Because the proton and electron are spinning distributions of electric charge they create minute magnetic fields which interact, creating a small energy difference between the state in which the poles are aligned versus counter-aligned. See the diagram to the left. This energy difference corresponds to the energy of radio waves at 21-centimeters.
Every once in a while (about once per 500 years) hydrogen atoms will collide, exciting an atom into the higher energy spin-aligned configuration. It will take as long as 30 million years for the atom to jump back to the lower energy state via a "spin-flip" emitting a 21 cm radio emission. The neutral hydrogen atom is distributed in clumpy fashion with cool, denser regions that astronomers call "clouds" but which are more like filaments. These regions have a typical temperature of about 100K and a density between 10 to 100 atoms per cubic centimeter (atom/cc). Surrounding the clouds is a warmer lower density medium with about 0.1 atom/cc and temperature (T) about 1000 K. On the other hand, there are billions and billions of hydrogen atoms in a typical cloud of gas. The result is that 21 cm radiation is a strong measure of the amount of hydrogen gas in various parts of the Milky Way. Top
Interstellar Dust
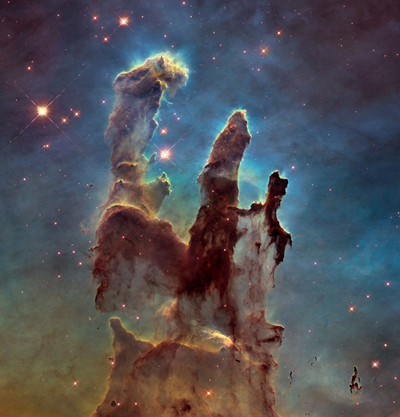
Interstellar dust is not like the dust that you might find under your refrigerator. It is made of very different materials. Interstellar dust particles are extremely small, just a fraction of a micron across, which happens to be approximately the wavelength of blue light waves. The particles are irregularly shaped, and are composed of silicates, carbon, ice, and/or iron compounds.
When light from other stars passes through the dust, a few things can happen. If the dust is thick enough, the light will be completely blocked, leading to dark areas. These dark clouds are known as dark nebulae. See the famous Pillars Of Creation nebula to the left.
Light passing through a dust cloud may not be completely blocked, although all wavelengths of light passing through will be dimmed somewhat. This phenomenon is known as "extinction".
Extinction is caused by light being scattered off of dust particles out of our line of sight, preventing the light from reaching us. The amount that the light is dimmed depends upon a few factors, including the thickness and density of the dust cloud, as well as the wavelength (color) of the incoming light.
Because of the size of the dust particles, scattering of blue light is favored. Therefore, less of the blue light reaches us, which means that the light that reaches us is more red than it would have been without the interstellar dust. This effect is known as reddening. (Note that this is not the same thing as redshift.) This process is similar to that make our sun so red at sunset.
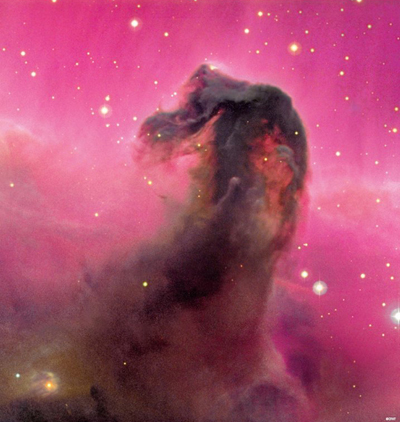
Although solid objects, dust grains are not immune to collisions. Collisions with high speed gas particles, UV photons and other grains will breakdown dust grains. For this reason, dust is only found in the cores of dark nebula where they are shielded from destructive effects.
Dust grains serve as sites for the formation of molecules and organic compounds. Their cold surfaces act as catalysts by allowing atoms to stick to cold grains so that there is enough time for a second atom to land, interact, and form a molecule. Collections of dust and molecular gases are called "molecular clouds".
Some dark nebula are are visible because an "emission nebula" lies behind them. These normally dark nebula are brightly illuminated from behind, such as the Horse Head Nebula shown at the left. This illumination happens often because the gas that is associated with the dark nebula can also be heated by nearby young stars causing it to glow as an emission nebula. (The Horse Head Nebula is also shown in infrared by the Hubble Telescope in the top banner above.)
The Horse Head Nebula is several tens of parsecs across and would envelop the whole local neighborhood of stars around our solar system. Stars seen in the image are foreground stars since the nebula is opaque. Top
Molecular Clouds
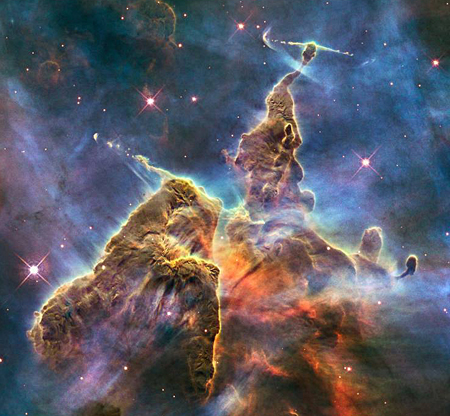
A molecular cloud is an accumulation of interstellar gas and dust. These clouds have very low temperatures of just 10 to 30 Kelvin and therefore the hydrogen is primarily composed of molecular hydrogen (H II). Compared to the ionized hydrogen in other areas of the interstellar medium, less than 1% of all hydrogen in the Milky Way is bound in molecular clouds. Pictured at the left is the famous Carina Nebula.
The size of these molecular clouds can vary from a few light years up to 600 light years and their total mass can reach several million solar masses. Molecular clouds with dimensions of more than about 15 light years are called Giant Molecular Clouds (GMCs).
Molecular clouds are so important because they are the raw material of stars and planets. A dark nebula is a very dense part of a bigger molecular cloud. These are the regions where new stars form.
These dense regions can only occur if the temperatures are very low, otherwise the thermal pressure of the gas leads to an expansion of the dark nebula and no new stars would be able to form. Molecular clouds consist mainly of hydrogen and dust particles which is all you need to form a new solar system.
Hundreds of different types of molecules have been detected in these clouds, among them water, ammonia, ethyl alcohol and even sugar and amino acids like glycine, the basic modules of life. More than 70 amino acids can be traced in meteorites. Meteorites represent the original composition of the molecular cloud that our solar system was formed out of. In most cases the basic meteorite structure has not changed much since the very first days of our solar system about 4.5 billion years ago.
These clouds do not last for a very long time. After new stars are born, their solar winds blow away the remaining cloud gas and dust. Only a fraction (about 10%) of the original material of the molecular cloud gets locked up in stars and planets. The rest of the material (90%) will be blown away into the interstellar medium and one day will be "recycled" into other molecular clouds. If one considers that for stars like our sun, approximately 50% of the hydrogen will also be returned to the interstellar medium. A total recycling rate of about 95% is achieved. In this sense, the universe is extremely efficient. Top
The Local Bubble
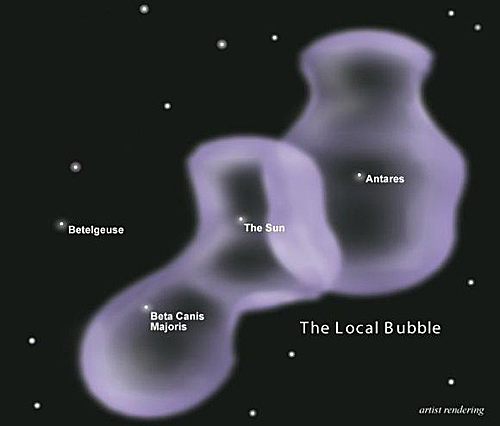
Decades of mapping the regional sky in soft x-rays had revealed a local glow, known as "The Local Bubble". The Local Bubble was discovered in the 1970s and 1980s. The Local Bubble is somewhat peanut-shaped, about 300 light years long, and filled with almost nothing. See the NASA artist's conception to the left.
Gas inside the bubble is very thin, approximately 0.001 particles per cubic centimeter, and very hot - roughly a million degrees. This is a a sharp departure from ordinary interstellar density and temperature. Evidence suggests that our solar system is moving through a region of space that may have been blasted totally clear by several supernova explosions during the past 20 million years. For more info about the Local Bubble, see The Cosmic X-Ray Background section.
However within the last decade, some scientists have been challenging the supernova interpretation, suggesting that much, if not all, of the soft x-ray background is instead a result of "charge exchange" with the solar wind. (See the Charge Exchange section.) To find out, an international team of researchers led by NASA developed an x-ray detector that could distinguish between the two possibilities.
On December 12, 2012, a NASA rocket, with the x-ray detector equipment aboard, reached a peak altitude of 160 miles and spent a grand total of five minutes above the earth's atmosphere. That was all the time it needed to measure the amount of "charge exchange" from the solar wind. The results clearly indicate that about 40 percent of the soft x-ray background originates from within the solar system. The 60 percent balance comes from the Local Bubble of hot gases, from the relics of ancient supernova explosions outside of our solar system, and possibly other interstellar sources. Top
The Local Cloud
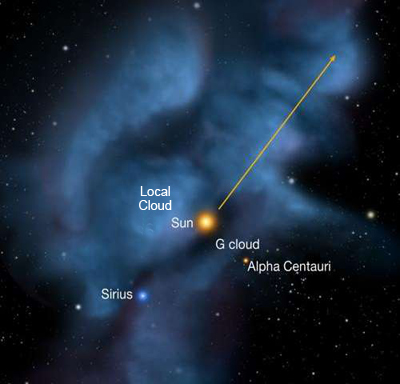
Our sun and the solar system are currently moving through a nearby cloud of interstellar gas called the "Local Cloud" which is also immersed in the "Local Bubble". See the NASA artist's illustration to the left. The Local Cloud is approximately 60 light years in diameter with our sun being approximately 4 light years from an edge. It contains a wispy mixture of hydrogen and helium atoms. The Local Cloud, features a density of 0.1 particles per cubic centimeter, and a temperature of about 6000 K,
Our sun and solar system are moving through the Local Cloud with a speed of about 50,000 miles per hour (23 km/sec.). As a result of our traveling motion, an interstellar wind of the same speed is blowing through our planetary system. This is similar to the wind one feels while driving a car with the top down. The observed temperature and density of the Local Cloud do not provide enough pressure to resist the crushing action of the hot gas from the Local Bubble. So how has the Local Cloud survived?
Voyager data showed that the Local Cloud is much more strongly magnetized than anyone had suspected - between 4 and 5 micro-gauss. This fairly strong magnetic field provides the extra pressure required to resist destruction from the Local Bubble. The Local Cloud is held at bay just beyond the edge of the solar system by the sun's magnetic field, the Heliosphere, which is inflated by the solar wind into a magnetic bubble more than 6 billion miles (10 billion km) wide.
The Heliosphere acts as a shield that protects the inner solar system from most outer space cosmic rays and interstellar clouds and bubbles. Also the Magnetosphere (the comet-like magnetic sphere around the earth) prevents highly charged fast-moving particles (mostly electrons and protons), propelled by the Solar Wind, Clouds and Bubbles, from hitting the earth. The Magnetosphere also does a good job of protecting us from Geo-Magnetic Storms from the sun. Top
The Gas Pile Up
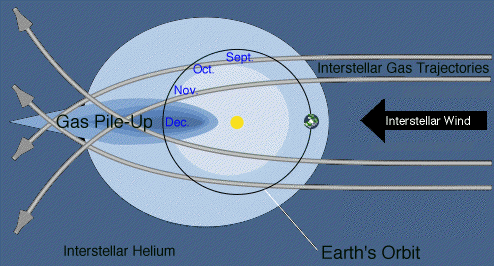
In the illustration to the left, note the interstellar Gas Pile-Up "downwind" due to the sun's gravity focusing the interstellar wind. As the earth revolves around the sun in the course of a year, satellites (most recently IBEX) have detected a gas pile up which peaks in the month of December as shown by a darker blue in the diagram. Satellites can determine the density of the medium by sampling how much neutral helium gas is found on any given day. It is heaviest in December and lightest in June when the earth is "upwind" of the Pile Up.
The sun sends out a solar wind in all directions, with speeds of 670,000 to 2,237,000 miles per hour (300 to 1000 km/sec). As mentioned in the section above, the interstellar wind is moving at a rather slow 50,000 miles an hour (23 km/sec.). One would think the gale force solar wind would just wipe out the interstellar wind. But that is not the case.
Typically, there is 0.1 atoms in each cubic centimeter of interstellar wind, and 10 ions in each cubic centimeter of solar wind (versus surface air which contains 3 x 10^19 molecules per cc). This means there is a great deal of dead space between the very tiny particles and collisions are rare. On average an interstellar gas atom can travel 100 times the distance between the sun and the earth before it is hit by a solar wind particle. The particles mostly just flow through each other without many collisions.